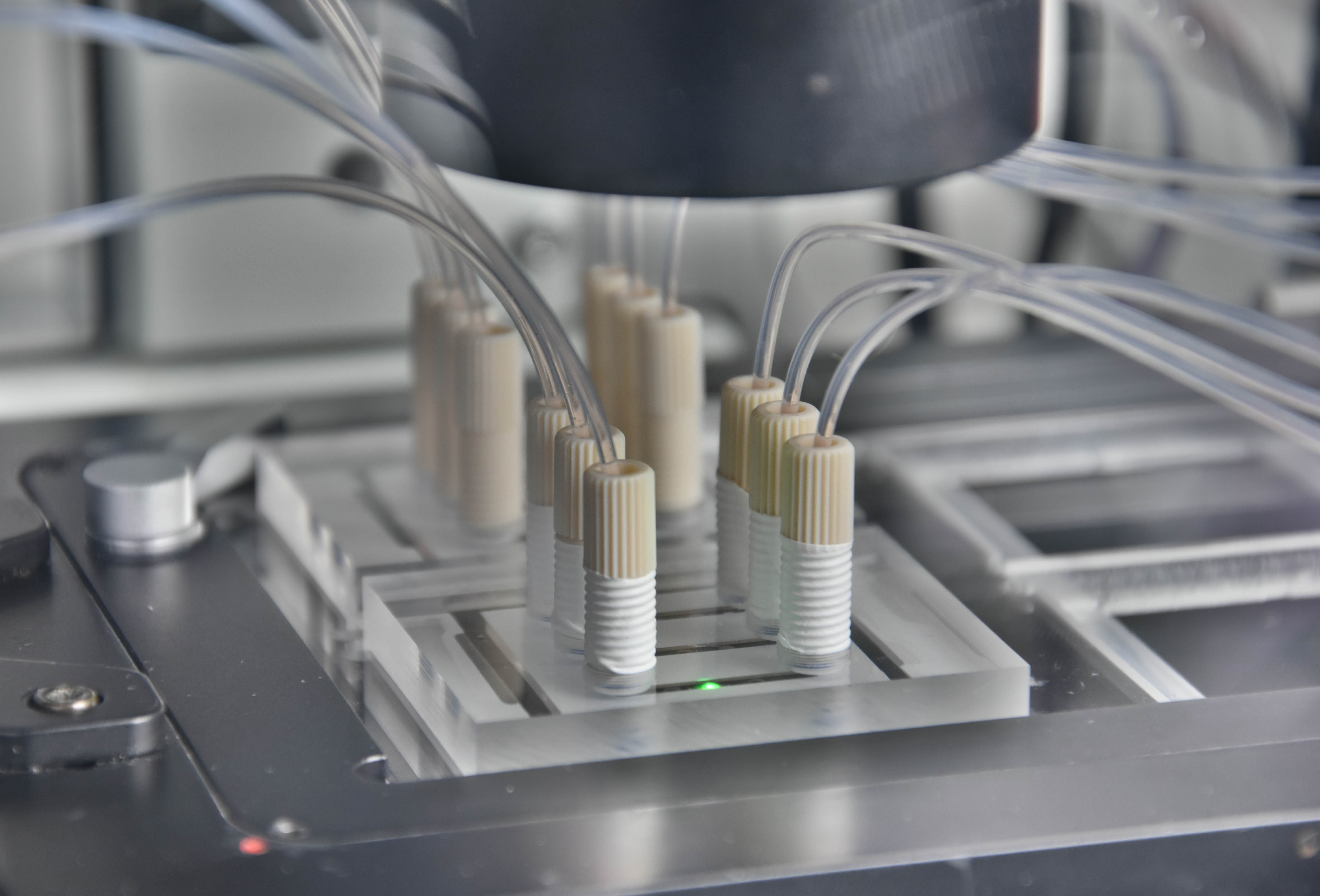
High-performance methods for the analysis of cells enable an always deeper insight into cell biological processes and thus form the basis for many medical and biotechnological applications. A wide range of methods for the cultivation and analysis of primary cells and cell lines is available at the institute. Cell culture laboratories equipped with state-of-the-art devices and microscopes of safety level S1 or S2 allow working with blood and many other biological cell samples. We develop customized procedures according to your individual requirements and specifications and support you in your preclinical research projects as well as drug or medical device testing.